IB Biology Nucleic Acids: Notes and Past Paper Question Examples
IB Biology Nucleic Acids: Notes and Past Paper Question Examples
7/19/20248 min read
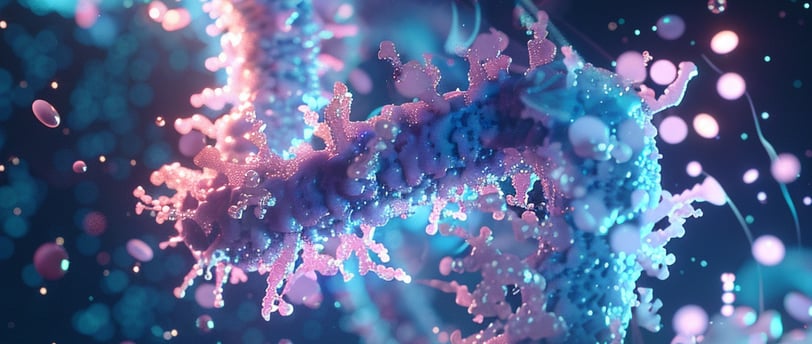
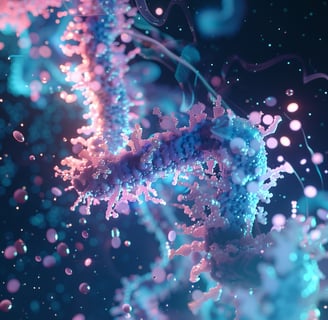
Introduction to Nucleic Acids
IB Biology Nucleic acids are fundamental biomolecules that serve as the blueprints for life. These complex macromolecules are essential for storing and transmitting genetic information in biological systems. There are two primary types of nucleic acids: deoxyribonucleic acid (DNA) and ribonucleic acid (RNA). Both play critical roles in cellular functions, heredity, and the expression of genetic traits.
The basic building blocks of nucleic acids are nucleotides, which are composed of three components: a phosphate group, a five-carbon sugar (deoxyribose in DNA and ribose in RNA), and a nitrogenous base. The nitrogenous bases are classified into two categories: purines (adenine and guanine) and pyrimidines (cytosine, thymine, and uracil). In DNA, the bases are adenine, guanine, cytosine, and thymine, whereas in RNA, thymine is replaced by uracil.
Nucleotides link together through phosphodiester bonds, forming long chains that constitute the backbone of the DNA or RNA molecule. In the case of DNA, these chains are arranged in a double helix structure, a discovery attributed to James Watson and Francis Crick. The double helix consists of two complementary strands running in opposite directions, held together by hydrogen bonds between paired bases: adenine with thymine and guanine with cytosine.
DNA's double helix structure is crucial for its function in storing genetic information. Each strand serves as a template for replication, ensuring accurate transmission of genetic information during cell division. RNA, on the other hand, is typically single-stranded and plays various roles, including acting as a messenger (mRNA), a structural component of ribosomes (rRNA), and a transporter of amino acids (tRNA) during protein synthesis.
Understanding nucleic acids is fundamental to grasping the molecular mechanisms of genetics. Their intricate structures and functions underscore the complexity and precision of biological systems, highlighting their indispensable role in the continuity of life.
DNA Structure and Function
The structure of DNA, or deoxyribonucleic acid, is a quintessential element in the study of molecular biology. Watson and Crick's pioneering work in 1953 introduced the double helix model, which serves as the foundation for our understanding of genetic material. This model illustrates that DNA is composed of two complementary strands that twist around each other, resembling a spiral staircase. Each strand is made up of nucleotides, which consist of a phosphate group, a deoxyribose sugar, and one of four nitrogenous bases: adenine (A), thymine (T), cytosine (C), and guanine (G).
The complementary base pairing is a critical feature of DNA structure. Adenine pairs with thymine through two hydrogen bonds, while cytosine pairs with guanine via three hydrogen bonds. These hydrogen bonds confer a significant degree of stability to the DNA molecule, ensuring the integrity of genetic information during cellular processes. Additionally, the antiparallel nature of the two DNA strands—where one strand runs 5’ to 3’ and the other 3’ to 5’—is crucial for the replication and transcription processes.
Functionally, DNA serves as the blueprint for protein synthesis through the processes of transcription and translation. During transcription, a specific segment of DNA is transcribed into messenger RNA (mRNA) by the enzyme RNA polymerase. This mRNA then migrates from the nucleus to the cytoplasm, where it serves as a template for protein synthesis. During translation, ribosomes read the mRNA sequence in sets of three nucleotides, known as codons, each of which codes for a specific amino acid. This sequence of amino acids then folds into a functional protein, which performs various tasks within the cell.
The genetic code, which dictates this translation process, is nearly universal among organisms, underscoring its evolutionary significance. It is composed of 64 codons, three of which are stop codons signaling the end of protein synthesis. The redundancy in the genetic code—where multiple codons can code for the same amino acid—adds a layer of protection against mutations, thereby preserving the organism's genetic integrity.
RNA Types and Functions
Ribonucleic acid (RNA) is crucial in the process of protein synthesis, playing various roles through its different types: messenger RNA (mRNA), transfer RNA (tRNA), and ribosomal RNA (rRNA). Unlike DNA, RNA contains ribose sugar instead of deoxyribose and utilizes uracil in place of thymine. These structural differences enable RNA to perform its unique functions within the cell.
mRNA (Messenger RNA)
mRNA serves as the intermediary between DNA and protein synthesis. During transcription, an mRNA strand is synthesized from the DNA template in the nucleus. This mRNA strand carries the genetic information encoded in DNA to the ribosomes, where translation occurs. The sequence of bases in mRNA determines the sequence of amino acids in the resulting protein, making mRNA a crucial component in gene expression.
tRNA (Transfer RNA)
tRNA is essential in translating the genetic code carried by mRNA into a sequence of amino acids. Each tRNA molecule has an anticodon region that is complementary to the codons on the mRNA strand. tRNA molecules transport specific amino acids to the ribosome, where they are added to the growing polypeptide chain. This ensures that the protein is synthesized according to the genetic instructions provided by the mRNA.
rRNA (Ribosomal RNA)
rRNA is a key structural and functional component of ribosomes, the cellular machines that assemble proteins. Together with proteins, rRNA forms the ribosome's core, facilitating the binding of mRNA and tRNA during translation. The rRNA catalyzes the formation of peptide bonds between amino acids, effectively acting as an enzyme to speed up the protein synthesis process.
In summary, the processes of transcription and translation are vital for gene expression and protein synthesis. During transcription, mRNA is synthesized from the DNA template, carrying genetic information to the ribosome. Translation then occurs, where tRNA and rRNA work in concert to decode the mRNA and assemble the corresponding amino acid sequence, resulting in the formation of functional proteins. These complex interactions highlight the indispensable roles of the different types of RNA in cellular biology.
DNA Replication and Repair
DNA replication is a critical process in cellular division, ensuring that genetic information is accurately transmitted from one generation to the next. This process is characterized by its semi-conservative nature, meaning that each new DNA molecule comprises one original (parental) strand and one newly synthesized strand. The semi-conservative model was confirmed through the Meselson-Stahl experiment, which demonstrated that DNA strands separate and serve as templates for new strand synthesis.
The replication process begins at specific sites known as origins of replication. In eukaryotic cells, there are multiple origins of replication to facilitate the copying of large amounts of DNA. Replication proceeds bidirectionally from each origin, forming replication forks that move outward, unwinding the DNA helix in both directions.
Several enzymes play crucial roles in DNA replication. Helicase unwinds the double helix, creating a replication fork. Single-strand binding proteins stabilize the unwound strands, preventing them from re-annealing. DNA polymerase then synthesizes the new DNA strand by adding nucleotides complementary to the template strand. However, DNA polymerase can only add nucleotides to an existing 3' end, which necessitates the presence of an RNA primer laid down by primase. The leading strand is synthesized continuously, while the lagging strand is synthesized discontinuously in short segments called Okazaki fragments. These fragments are later joined together by DNA ligase, ensuring a continuous DNA strand.
Maintaining genetic integrity during replication is paramount, and cells employ various DNA repair mechanisms to correct errors that may arise. DNA damage can result from environmental factors such as UV radiation, chemicals, and reactive oxygen species, or from errors during replication. Common types of DNA damage include base mismatches, thymine dimers, and single or double-strand breaks.
To mitigate these errors, cells utilize several repair processes. Mismatch repair corrects errors that escape the proofreading activity of DNA polymerase. Nucleotide excision repair removes bulky lesions like thymine dimers, while base excision repair addresses small, non-helix-distorting base lesions. For more severe damage, such as double-strand breaks, cells use homologous recombination or non-homologous end joining to restore DNA integrity.
Mutations and Genetic Variability
Mutations are alterations in the DNA sequence that can occur in various forms, including point mutations, insertions, deletions, and chromosomal alterations. Point mutations involve a change in a single nucleotide base pair, which can lead to a silent, missense, or nonsense mutation, depending on the impact on the resulting protein. Insertions and deletions, also known as indels, entail the addition or loss of nucleotide bases in the DNA sequence, potentially causing frameshift mutations that alter the reading frame of the gene. Chromosomal alterations, such as duplications, inversions, translocations, and aneuploidies, affect larger segments of DNA and can have profound consequences on an organism's genome.
Mutations can arise spontaneously due to errors in DNA replication or be induced by environmental factors such as ultraviolet radiation, chemicals, or viruses. Spontaneous mutations are generally rare events but can accumulate over time, contributing to genetic diversity within populations. Environmental mutagens increase the mutation rate, potentially leading to greater variability but also to detrimental effects if critical genes are affected.
The impact of mutations on protein function and organismal traits varies widely. Some mutations may be neutral, having no significant effect on the organism, while others can be beneficial or deleterious. Beneficial mutations may confer an advantage to the organism, enhancing its survival and reproductive success. Conversely, deleterious mutations may reduce fitness, potentially leading to genetic disorders or decreased viability.
Mutations play a crucial role in genetic diversity and evolution. They introduce new genetic variations into populations, which can be acted upon by evolutionary forces such as gene flow, genetic drift, and natural selection. Gene flow involves the transfer of genetic material between populations, promoting genetic diversity and potentially introducing beneficial traits. Genetic drift refers to random changes in allele frequencies within a population, which can lead to significant genetic shifts over time, especially in small populations. Natural selection, the differential survival and reproduction of individuals based on their genetic traits, drives the adaptation of populations to their environments, with advantageous mutations becoming more common over generations.
Past Paper Question Examples
One of the most effective ways to prepare for the IB Biology exam is by practicing with past paper questions. This section provides a collection of questions related to nucleic acids, complete with detailed answers and explanations. These examples are designed to help you understand how nucleic acids topics are tested, and to provide strategies for effectively answering such questions.
Question 1
Question: Describe the structure of DNA and explain the significance of its double-helix configuration. (8 marks)
Answer: DNA (deoxyribonucleic acid) is composed of two long strands of nucleotides that form a double helix. Each nucleotide consists of a phosphate group, a deoxyribose sugar, and a nitrogenous base (adenine, thymine, cytosine, or guanine). The two strands are antiparallel, running in opposite directions, and are held together by hydrogen bonds between complementary bases: adenine pairs with thymine, and cytosine pairs with guanine. The double-helix structure is significant because it allows for the replication of DNA and the accurate transmission of genetic information. During replication, the two strands separate, and each serves as a template for the formation of a new complementary strand, ensuring that genetic information is accurately copied.
Explanation: This question tests your understanding of the molecular structure of DNA and its biological importance. To score well, you need to describe the components of DNA and the base-pairing rules clearly. Additionally, you should explain why the double-helix configuration is crucial for DNA replication. Focus on providing a detailed and coherent explanation, as this will demonstrate your comprehensive understanding of the topic.
Question 2
Question: Explain the role of mRNA in protein synthesis. (6 marks)
Answer: Messenger RNA (mRNA) plays a crucial role in protein synthesis by serving as a template for the sequence of amino acids in a protein. The process begins with transcription, where a segment of DNA is used to produce an mRNA molecule. This mRNA then exits the nucleus and enters the cytoplasm, where it associates with a ribosome. During translation, the ribosome reads the mRNA sequence in sets of three bases, known as codons. Each codon specifies a particular amino acid, which is brought to the ribosome by transfer RNA (tRNA). The ribosome assembles the amino acids in the correct order to form a polypeptide chain, which folds into a functional protein.
Explanation: This question focuses on your ability to explain the role of mRNA in the central dogma of molecular biology. Ensure you clearly outline the steps involved in transcription and translation, and the specific function of mRNA in these processes. Highlighting the flow of genetic information from DNA to protein will help you construct a thorough and well-organized answer.
Exam Tips
To excel in the IB Biology exam, particularly in sections related to nucleic acids, consider the following tips:
Time Management: Allocate your time wisely. Spend a few minutes reading through all the questions before starting. Prioritize questions you are most confident in and ensure you leave time to review your answers.
Understanding the Question: Carefully read each question to understand what is being asked. Look for key terms and instructions, such as "describe," "explain," or "compare."
Avoid Common Pitfalls: Avoid vague or overly brief answers. Provide detailed explanations and, where applicable, use diagrams to support your answers. Ensure accuracy in terminology and avoid common misconceptions, such as confusing RNA with DNA.
By practicing with past paper questions and applying these strategies, you can improve your understanding of nucleic acids and enhance your performance in the IB Biology exam.
#1 IB Tutors in Hong Kong
Leading IB tutors in HK with unmatched experience and success rates.
Contact
Join
+852 66947789
© 2024. All rights reserved.